Gas-Solid Interactions Research in the Sibley School
Beside our experiments on circulating fluidization, we have also studied the interactions of gases and agitated solids. Typically, we consider solids that are sufficiently agitated to affect the surrounding gas, but that are too massive to be moved significantly by the gas. We have considered fluid mechanics and heat transfer in dense suspensions of gases and agitated solids, with applications to the pneumatic transport of massive particles.
A challenge to theory is the formation of particle clusters. The following article points to recent progress by Mitrano et al (2014) on the subject of clusters: M.Y. Louge: "The surprising relevance of a continuum description to granular clusters," J. Fluid Mech. 742, 1-4 (2014), doi:10.1017/jfm.2013.650.
Gas-solid suspension results
Our main publications on this subject include:
H. Xu, R. Verberg, D.L. Koch and M.Y. Louge: "Dense, bounded shear flows of agitated solid spheres in a gas at intermediate Stokes and finite Reynolds numbers," J. Fluid Mech. (2009), vol. 618, pp. 181–208.
In this paper, we describe a theory capturing the Lattice-Boltzmann numerical simulations of Verberg and Koch (2006) for massive particles sheared in the presence of an interstitial gas between two bumpy boundaries. We used these results to predict heat transfer enhancement in agitated suspensions.
Lattice-Boltzmann simulations of Verberg and Koch.
The movie shows spherical grains and gas velocity streaklines around
them.
(Chris Pelkie rendered the simulation).
Louge, M.Y., Mastorakos, E. and Jenkins, J.T.: “The Role of Particle Collisions in Pneumatic Transport,” J. of Fluid Mech. 231, 345-359 (1991).
In this paper, we analyze the steady, fully-developed flow of
massive particles (i.e., large Stokes numbers) suspended in turbulent
gas in a vertical pipe. In this regime, the agitation of the particles
is set by binary collisions among grains, rather than interactions with
fluctuation velociy of the gas. We test the theory against the data of
Tsuji, et al (1984).
An excerpt of Louge, Mastorakos and Jenkins (1991)
is available here.
H. Xu: "Collisional granular flows with and without gas interactions in microgravity," Ph.D. thesis, Cornell University (2003).
Haitao Xu's Ph.D. thesis contains information on boundary conditions, granular segregation and gas-solid interactions.
Heat transfer results
X. Chen and M.Y. Louge: “Heat transfer enhancement in dense suspensions of agitated solids. Part I: Theory.”, International J. Heat Mass Transfer, 51 (2008) 5108–5118.
This paper outlines a new theory for heat transfer enhancement through dense homogeneous suspensions of agitated solids in conductive fluids. The theory couples the fluid and solid phases through a volumetric source term. The enhancement is governed by a Damkohler number demarcating an "exchange limit" where the source term dominates, and a "diffusion limit" set by the ability of agitated particles to self-diffuse. We point out effects of particle ordering on mixture conductivity and volumetric heat exchange rate, carry out thermal simulations to justify the form of these terms, and model further enhancements from gas velocity fluctuations induced by solids of high agitation.
X. Chen and M.Y. Louge: “Heat transfer enhancement in dense suspensions of agitated solids. Part II: Experiments in the exchange limit.”, International J. Heat Mass Transfer, 51 (2008) 5119–5129.
We test the theory of Part I in the exchange limit by vibrating acrylic and aluminum spheres in a box consisting of two flat, vertical isothermal walls, two bumpy, horizontal, insulated walls, and two flat vertical insulated surfaces. The steady heat flux through the thermally-guarded hot wall is recorded at different temperatures of the opposite wall cooled by thermoelectric modules, and enhancements of suspension conductivity are calculated using a lumped-parameter model of the box. To compare results and theory, we also predict vertical profiles of agitation and solid volume fraction in the box using granular dynamics.
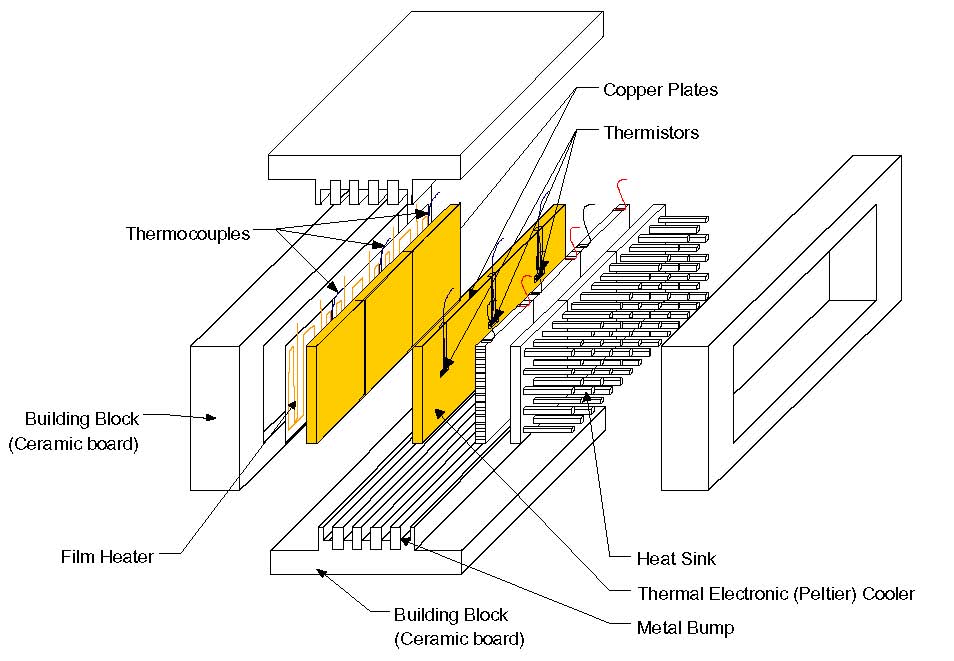
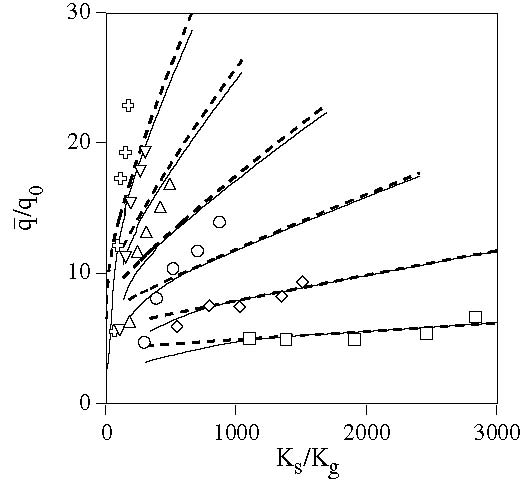
M.Y. Louge and X. Chen: “Heat transfer enhancement in dense suspensions of agitated solids. Part III: Thermophoretic transport of nanoparticles in the diffusion limit.”, International J. Heat Mass Transfer, 51 (2008) 5130–5143.
This paper extrapolates the diffusion limit discussed in Part I to suspensions of nanoparticles in a liquid. We take a sceptical view of the exceptional heat transfer enhancements that have been reported in the literature.
Because nanoparticles are agitated by Brownian motion, their self-diffusivity is modest, the fluid and solid phases share the same temperature, and mixture theory should predict the effective suspension conductivity. We show how thermophoresis creates suspension inhomogeneities, suggest ways to mitigate the latter with ultrasonic forcing, and examine consequences on heat transfer. To inform a debate on nanofluids heat transfer, we show that anomalous conductivity enhancements reported with hot-wire thermal conductimetry can be an experimental artifact of thermophoretic migration along the temperature gradient or timing in the observations.
We provide zipped files containing Matlab, Mathematica and Excel documents that we used to carry out computations for Part I, Part II and Part III of Chen and Louge (2008). These programs include predictions as diverse as the non-linear behavior of a 1-D vibrated granular system based on the kinetic theory of granular gases, the spatial distribution function of spheres at a flat wall, the thermophoresis of nanoparticles, transient solutions for hot-wire conductimetry, ultrasonic forcing of nanofluids, and more. For explanations, see the readme text file first; then look at comments in the corresponding programs.
Louge, M., Mohd. Yusof J. and Jenkins J.T.: “Heat Transfer in the Pneumatic Transport of Massive Particles,” Int. J. Heat & Mass Trans. 36 (2), 265-275 (1993).
In this paper, we analyze the steady, fully-developed heat transfer
to the walls of a vertical pipe from a dilute turbulent suspension of
massive particles in a gas. We exploit the theory of Louge, Mastorakos and Jenkins
for fluid dynamics of the suspension, and we write coupled equations
for the thermal energies of the particle and gas phase. We test the
theory against data from Jepson, et al. This paper is a precursor of
the more recent theory of Chen
and Louge for suspensions of agitated grains in a gas.
An excerpt of Louge, Mohd. Yusof and Jenkins (1993)
is available here.
Microgravity experiments
NASA-Glenn Research Center sponsored our project SiGMA (Solids
Interacting with a Gas in a Microgravity Apparatus), which was part of
the Granular Flow Module on the International Space Station, from 1998
to 2005. The final report is shown below. We successfully tested our own prototype
of the facility on NASA's KC-135 microgravity aircraft. A test matrix
of experiments that we had planned for the Space Spation is found in Haitao Xu's PhD thesis.
M. Y. Louge, J.T. Jenkins and A. Reeves, “Studies of Gas-Particle Interactions in a Microgravity Flow Cell,” NASA Contract NAG3-2705, final report, March 2007.