Gas-solid Heat Transfer Research at Cornell
We have carried out research on heat transfer in gas-solid
suspensions and agitated granular media. The principal results appear
in the publications below.
Results
X. Chen and M.Y. Louge: “Heat transfer enhancement in dense suspensions of agitated solids. Part I: Theory.”, International J. Heat Mass Transfer, 51 (2008) 5108–5118.
This paper outlines a new theory for heat transfer enhancement through dense homogeneous suspensions of agitated solids in conductive fluids. The theory couples the fluid and solid phases through a volumetric source term. The enhancement is governed by a Damkohler number demarcating an "exchange limit" where the source term dominates, and a "diffusion limit" set by the ability of agitated particles to self-diffuse. We point out effects of particle ordering on mixture conductivity and volumetric heat exchange rate, carry out thermal simulations to justify the form of these terms, and model further enhancements from gas velocity fluctuations induced by solids of high agitation.
X. Chen and M.Y. Louge: “Heat transfer enhancement in dense suspensions of agitated solids. Part II: Experiments in the exchange limit.”, International J. Heat Mass Transfer, 51 (2008) 5119–5129.
We test the theory of Part I in the exchange limit by vibrating acrylic and aluminum spheres in a box consisting of two flat, vertical isothermal walls, two bumpy, horizontal, insulated walls, and two flat vertical insulated surfaces. The steady heat flux through the thermally-guarded hot wall is recorded at different temperatures of the opposite wall cooled by thermoelectric modules, and enhancements of suspension conductivity are calculated using a lumped-parameter model of the box. To compare results and theory, we also predict vertical profiles of agitation and solid volume fraction in the box using granular dynamics.
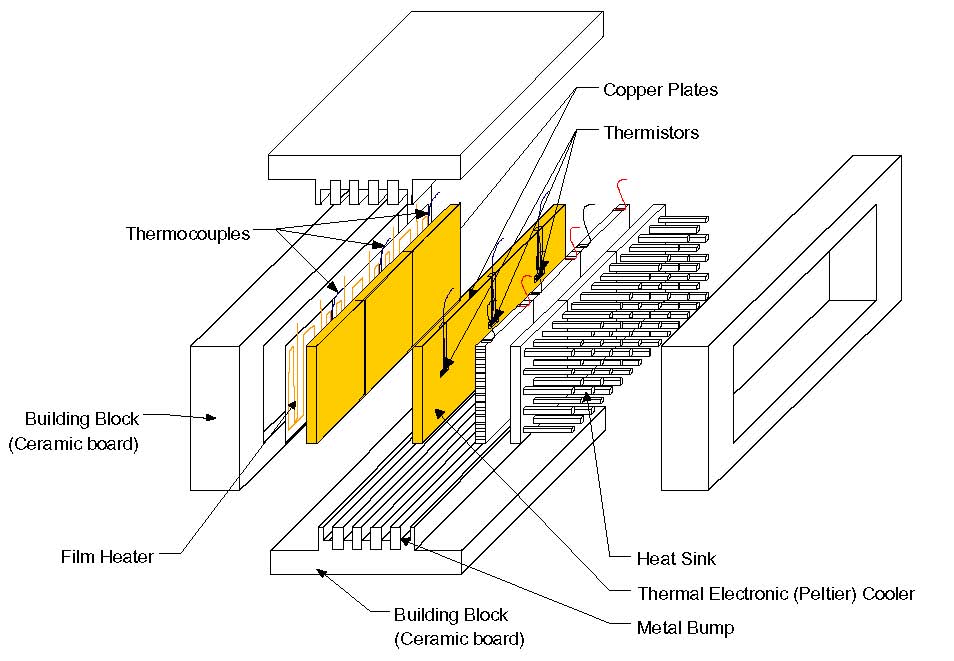
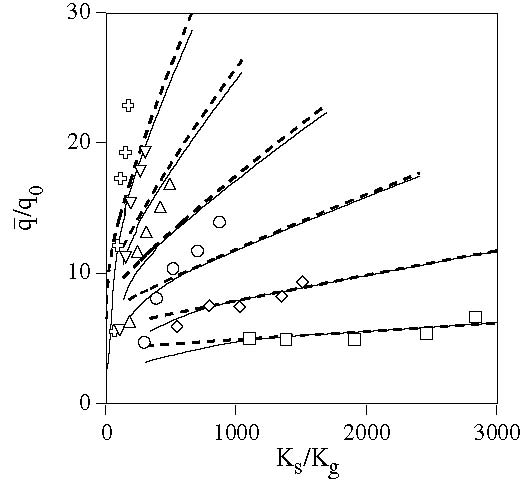
M.Y. Louge and X. Chen: “Heat transfer enhancement in dense suspensions of agitated solids. Part III: Thermophoretic transport of nanoparticles in the diffusion limit.”, International J. Heat Mass Transfer, 51 (2008) 5130–5143.
This paper extrapolates the diffusion limit discussed in Part I to suspensions of nanoparticles in a liquid. We take a skeptical view of the exceptional heat transfer enhancements that have been reported in the literature.
Because nanoparticles are agitated by Brownian motion, their self-diffusivity is modest, the fluid and solid phases share the same temperature, and mixture theory should predict the effective suspension conductivity. We show how thermophoresis creates suspension inhomogeneities, suggest ways to mitigate the latter with ultrasonic forcing, and examine consequences on heat transfer. To inform a debate on nanofluids heat transfer, we show that anomalous conductivity enhancements reported with hot-wire thermal conductimetry can be an experimental artifact of thermophoretic migration along the temperature gradient or timing in the observations.
We provide zipped files containing Matlab, Mathematica and Excel documents that we used to carry out computations for Part I, Part II and Part III of Chen and Louge (2008). These programs include predictions as diverse as the non-linear behavior of a 1-D vibrated granular system based on the kinetic theory of granular gases, the spatial distribution function of spheres at a flat wall, the thermophoresis of nanoparticles, transient solutions for hot-wire conductimetry, ultrasonic forcing of nanofluids, and more. For explanations, see the readme text file first; then look at comments in the corresponding programs.
A. Elizabeth Griffith, M.Y. Louge and Jamaludin Mohd. Yusof: “Simultaneous, Non-invasive Measurements of Convective Heat Transfer and Solid Volume Fraction at the Wall of an Entrained Gas-Solid Suspension,” Rev. Sci. Instrum. 71, 2922-2927 (2000).
In this paper, we describe a constant-temperature heat flux probe
fitted within the central grounded surface of a capacitance probe. This
allowed us to conduct simultaneous
measurements of solid volume fraction and heat transfer coefficient at
the wall of vessel holding a gas-solid suspension.
An excerpt of Griffith, Louge and Mohd.
Yusof (2000) is available here.
A. Elizabeth Griffith: “Convective heat transfer scaling at the wall of circulating fluidized bed risers” Ph.D. thesis (2000).
Elizabeth Griffith describes and interpret experiments in our
circulating fluidized bed facilities, in which she correlates the
presence of particle clusters with heat transfer coefficients at the
riser wall. She uses the instrument combining a wall capacitance probe
and a small constant-temperature heat flux probe described by Griffith, et al (2000). She also uses a unique thermal marking technique to record the residence length of clusters at the wall.
Louge, M., Mohd. Yusof J. and Jenkins J.T.: “Heat Transfer in the Pneumatic Transport of Massive Particles,” Int. J. Heat & Mass Trans. 36 (2), 265-275 (1993).
In this paper, we analyze the steady, fully-developed heat transfer
to the walls of a vertical pipe from a dilute turbulent suspension of
massive particles in a gas. We exploit the theory of Louge, Mastorakos and Jenkins
for fluid dynamics of the suspension, and we write coupled equations
for the thermal energies of the particle and gas phase. We test the
theory against data from Jepson, et al. This paper is a precursor of
the more recent theory of Chen
and Louge for suspensions of agitated grains in a gas.
An excerpt of Louge, Mohd. Yusof and Jenkins (1993)
is available here.