Research on eruption currents in the Sibley School
One of the most dramatic example of eruption currents is the frontal region of a powder snow avalanche, as shown below. An article by Betty Sovilla, Jim McElwaine and Michel Louge delineates its principal regions. Links to papers mentioned in this figure are below.
Our work on eruption currents was funded by the ACS Petroleum Research Fund and was a collaboration with Barbara Turnbull at the
University of Nottingham and Edwin (Todd) Cowen at Cornell. It is the subject of the thesis of Cian Carroll.
Eruption currents are driven by massive frontal entrainment. An example is powder snow avalanches. Although these phenomena resemble gravity currents generated as a heavier fluid is released into a lighter one (for example following a dam break), their fluid mechanics is radically different. This is why we coined the term "eruption currents" to describe them.
In the summer 2014, Michel Louge spent time as a Distinguished Visiting Fellow of the Royal Academy of Engineering with Dr. Barbara Turnbull at the University of Nottingham. They worked on extending their eruption current models to deep-sea sediment slides, such as the 1929 Grand Banks tsunami, which threaten offshore oil infrastructure.
As background for this study, we provide here the original paper and a translation from the original Russian
(courtesy of Victor Kozlov and Stanislav Sovetov of Perm State
Humanitarian Pedagogical University) of seminal observations in powder
snow avalanches near Mount Elbrus in the Caucasus mountains by Grigorian,
S. S., N. A. Urumbiev, and I. V. Nekrasov (1982), Data of geological
studies (in Russian), Academy of Sciences of the U.S.S.R. Section of
Glaciology of the Soviet Geophysical Committee and Institute of
Geography, 44, 87–94. This paper is not easily available otherwise.
Visualize Michel Louge's presentation on the "Role of pore pressure gradients in geophysical flows over permeable substrates" at the Kavli Institute of Theoretical Physics in Santa Barbara during the conference on Fluid-Mediated Particle Transport in Geophysical Flows on Wednesday, December 18, 2013. The first part of the presentation concerns powders snow avalanches. The second discusses the role of the porous sand bed in desert ripples.
Results
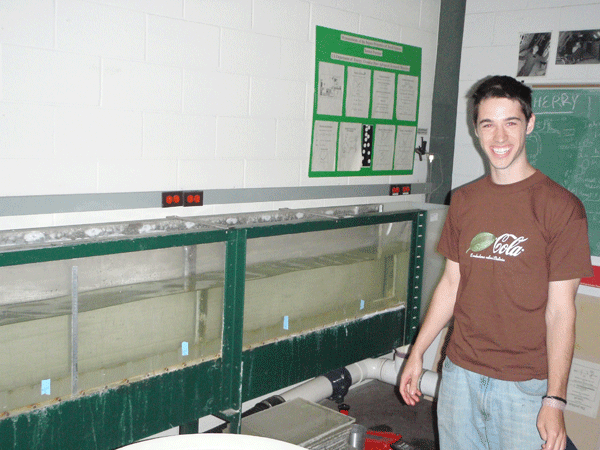
Cian Carroll in front of the flume.
Louge, M. Y., C.S. Carroll, and B. Turnbull (2011), Role of pore pressure gradients in sustaining frontal particle entrainment in eruption currents -- the case of powder snow avalanches, J. Geophys. Res. 116, F04030, 13 PP. (2011) doi:10.1029/2011JF002065.
Our work is relevant to the formation of
powder snow avalanches. In this manuscript published in the J. of
Geophysical Research,
we present a model that underscores the role played by the porous snow
cover in sustaining large, rapid, dilute powder avalanches over weakly
cohesive snow.
The model attributes massive localized
material injection into the avalanche head to synergistic pressure
gradients established within the porous cover by the very static
pressure field that this influx induces along the pack surface.
Treating massive frontal snow entrainment as a source of fluid, we show
that static pressure time-histories recorded at the Vallee de la Sionne (Switzerland) by Turnbull and McElwaine with the WSL Institute for Snow and Avalanche Research SLF conform to the classical Rankine half-body flow field.
We calculate pore pressure within the snow
cover and, from the resulting pressure gradient, find stresses on a
vertical failure plane. After inferring an upper bound for snow
cohesion from pressure records, we derive a sufficient condition for
steady failure that sets the plowing depth, through which the cover
changes from porous solid to suspension. Fluidization of the cover's
top surface imposes another relation among maximum density, internal
friction and cohesion of the pack, maximum cloud size and minimum
avalanche speed. Altogether, these conditions dictate which snow covers
can produce powder snow avalanches.
Once fluidized, about 10 to 20% of the plowed snow pack is ejected into the powder cloud to feed the avalanche. The calculation of this ratio will appear in another paper, see below, in which we model the dynamics of the head of powder avalanches.
Laboratory experiments conducted by Roche
et al recently showed that a depression does exist in the porous medium
behind the front. Such depression could fluidize the medium and provide
a source of material to the gravity current overhead. See Roche,
O., S. Montserrat, Y. Nino, and A. Tamburrino (2010), Pore fluid
pressure and internal kinematics of gravitational laboratory
air‐particle flows: Insights into the emplacement dynamics of
pyroclastic flows, J. Geophys. Res., 115, B09206,
doi:10.1029/2009JB007133.
Perry Bartelt and Othmar Buser asked pertinent questions on the theory that we presented in the above JGR 2011 article. See Bartelt,
P., and O. Buser (2012), Powder cloud eruptions—Where is the air
reservoir and blow-out motor in the Rankine half-body? Comment on “Role
of pore pressure gradients in sustaining frontal particle entrainment
in eruption currents: The case of powder snow avalanches” by M. Y.
Louge et al.
We replied with the following clarification:
Louge, M. Y., C. S. Carroll, and B. Turnbull (2012), Reply to comment by P. A. Bartelt and O. Buser on “Role of pore pressure gradients in sustaining frontal particle entrainment in eruption currents: The case of powder snow avalanches”, J. Geophys. Res., 117, F02016, doi:10.1029/2012JF002380.
C.S. Carroll, B. Turnbull, and Louge, M. Y., (2012), Role of fluid density in shaping suspension currents driven by frontal particle blow-out, Phys. Fluids 24, 066603 (2012).
We study the role of suspension density in eruption currents, a regime of gravity-driven flow that is sustained by massive, localized blow-out of particles acting as a steady source of heavier fluid injected into a uniform flow at high Reynolds number. Inspired by the potential flow solution of Saffman and Yuen [J. Fluid Mech. 123, 459 (1982)], we show that the relative density difference between the two fluids swells the size of the current's head without changing its shape, while inducing a velocity jump at the interface. We test this inviscid theory against inviscid and large-eddy-simulations (LES). We also conduct experiments in a water flume, where a line source of fluorescent brines of various densities is injected in a cross-stream and visualized with a narrow sheet of light. Simulations and experiments reveal that, with isotropic velocity distribution on a finite source, eruption currents expand further and develop interface oscillations, but that the inviscid theory still captures relative swelling induced by density. We compare predictions to the static pressure data of McElwaine and Turnbull [J. Geophys. Res. 110, F03010 (2005)] in powder snow avalanches.
This animation of a 2D LES numerical simulations shows how the velocity profile at the source creates oscillations at the cloud interface in viscous fluids. Using these simulations, we show in the above paper that air entrainment at the head of powder snow cloud is small, but that it is significant in the tail.
This Matlab file calculates the solution above the mean interface using a collocation technique similar to Saffman and Yuen [J. Fluid Mech. 123, 459 (1982)]. This document discusses accuracy of the method and its limitations at large Ri. In particular, ar large radial coordinate, the infinite series do not always converge at large Ri.
Photograph of the experiment showing flume, source nozzle, light sheet and fluorescent source fluid illuminated by the light sheet. In this configuration, the nozzle tube casts an inevitable shadow region that we exclude from image analysis.
Time-averaged ensemble of 500 frames with false color indicating fluorescein concentration in ppm.
Gradient image with superimposed separatrix fit.
C.S. Carroll, M.Y. Louge, and B. Turnbull (2013), Frontal dynamics of powder snow avalanches, J. Geophys.
Res. 118, 1–12, doi:10.1002/jgrf.20068.
We analyze frontal dynamics of dilute powder snow avalanches sustained by rapid blow-out behind the front. Such material injection arises as a weakly cohesive snow cover is fluidized by the very pore pressure gradient that the particle cloud induces within the snowpack. We model cloud fluid mechanics as a potential flow consisting of a traveling source of denser fluid thrust into a uniform airflow. Stability analysis of a mass balance involving snow cover and powder cloud yields relations among scouring depth, frontal height, speed, mixed-mean density and impact pressure when the frontal region achieves a stable growth rate. We compare predictions with field measurements, show that powder clouds cannot reach steady frontal speed on a uniform snowpack with constant cloud width, and derive a criterion for cloud ignition. Because static pressure is continuous across the mean air-cloud interface and deviatoric stresses are negligible, frontal acceleration is insensitive to local slope, but instead arises from a deficit of flow-induced suction in the wake. We calculate how far a powder cloud travels until its frontal mixed-mean density becomes stable, and show how topographic spread can hasten its collapse.
C.S. Carroll, M.Y. Louge, and B. Turnbull (2013), Supplementary online material for Frontal dynamics of powder snow avalanches, J. Geophys. Res. 118, 1–12, doi:10.1002/jgrf.20068.
Download our presentation to the EGU meeting in Vienna, April 22-27, 2012. (Creative Commons Attribution 3.0 License)
Download our slides for the ICACM-2013 conference in Aussois,
May 2013. This presentation draws attention to the role of pressure
gradients in counteracting gravity in porous media such as snowpacks
and desert sand ripples.
M.Y. Louge, B. Turnbull and C.S. Carroll (2012), Volume growth of a powder snow avalanche, Annals of Glaciology 53(61), doi:10.3189/2012AoG61A030.
We contrast the frontal dynamics of dilute powder snow avalanches with the behavior of their tail. While the former can be regarded as a fast-moving eruption current dominated by synergistic material injection into a short head, the latter behaves as a nearly arrested dilute cloud of particles expanding by progressive incorporation of ambient air, or by entrainment of snow cover material by late avalanches trailing well behind the front.
Betty Sovilla, Jim N. McElwaine, and Michel Y. Louge (2015) The structure of powder snow avalanches (Structure des avalanches en aerosol), Compte-Rendus de Physique 16, http://dx.doi.org/10.1016/j.crhy.2014.11.005.
Powder snow avalanches (PSAs) can be hundreds of metres high and descend at astonishing speeds. This review paints a composite picture of PSAs from data acquired at the Vallee de la Sionne test site in Switzerland, including time-histories of snow cover thickness from buried radar and, at several elevations on a pylon, impact pressures from load cells, air pressure, particle velocity from optical sensors, and cloud density and particle cluster size from capacitance probes. PSAs feature distinct flow regions with stratification in mean density. At the head, highly fluctuating impact pressures weaken with elevation, while vertical velocity profiles evolve rapidly along the flow, suggesting that surface snow layers of light, cold, cohesionless snow erupt into a turbulent, inhomogeneous, recirculating frontal cloud region. For hundreds of metres behind the head, cloud stratification sharpens with the deposition of suspended cloud particles, while a denser basal flow of increasing thickness forms as deeper, warmer and heavier parts of the weakened snow cover are entrained. Toward the tail, vertical velocity profiles are more uniform, impact pressures become lower and steadier as the flow becomes thinner, and snow pack entrainment is negligible.
Valance, A. and M. Louge (2015) Granular physics (Physique des milieux granulaires), Comptes Rendus Physique 16, doi:10.1016/j.crhy.2014.11.007.
This article prefaces a special issue on granular physics guest-edited by Alexandre Valance and Michel Louge for Comptes-Rendus Physique, the journal of the French Academy of Sciences.